Cindy Regal Devises New Tricks To Measure The Quantum World
Cindy Regal builds stuff. She designs it, constructs it, troubleshoots it. It’s a practice she’s known and loved as early as her middle school years in Duluth, Minnesota, where she crafted a clock in woodshop class. In college, she was taking part in research to build lasers. These days, she develops and studies tiny devices that could serve as extremely precise sensors—from nanometer-thin membranes that obey the rules of quantum mechanics, to single atoms held in a laser beam.
“I like the process of building something creative to measure something new,” the University of Colorado, Boulder physicist says.
She uses her creations to interact with the physical world in novel ways, in pursuit of measurements that might reveal truths about nature or inspire technology. “I really like being an experimentalist,” she says. “I like to be able to make something and get feedback from the real world.”
Regal spoke to 1400 Degrees about her career, from discovering a new state of matter in graduate school to her current experiments developing the hardware for quantum technologies.
How did you end up studying physics?
In undergrad, I was in between picking chemistry or physics. I did a research experience for undergraduates program in chemical physics at the University of Oregon, with both chemists and physicists. I was into the laser spectroscopy aspect of the lab, and that was exciting. I liked how you could build something that ultimately allowed you to learn something.
You were part of the team that made the first ultracold fermionic condensate in 2003, when you were a grad student at the University of Colorado, Boulder. What is that?
Fermions are spin one-half particles, like electrons, or in the case of our experiment, potassium-40 atoms. According to the Pauli exclusion principle, no two fermions can occupy the same quantum state. They tend to repel each other by stacking up in energy levels instead. But under special circumstances, they can pair up to form particles with integer spin, which are bosons. Bosons and fermions are opposites in many ways. Bosons like to bunch together into the same spatial location. For my PhD, I got pairs of fermions to behave like bosons. The fermion pairs formed a state of matter known as a condensate for the first time.
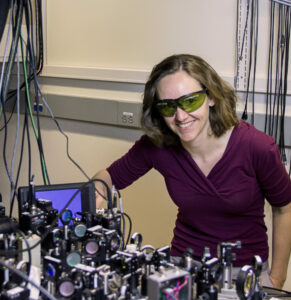
What was the difficulty in making the condensate?
The key challenge was to make the particles in the gas interact in the way we wanted. Ordinarily, the atoms repel each other. But using a magnetic field, you can engineer a situation known as a Feshbach resonance that changes the repulsion into an attraction.
One turning point for me was when I was trying to make molecules from the atoms. I wondered if I could confirm I’d made molecules by breaking pairs of atoms and seeing how much energy is released. So I tried it! Using a certain type of spectroscopy, I saw that the fermionic atoms had bonded into molecules. That was one of the first times I felt ownership of an experiment. It made me more confident to build things just to explore.
Why was this experiment a big deal?
These fermionic condensates can mimic the physics of superconductors. Both the condensate and the superconductor consist of fermion pairs that bond weakly to each other to form what’s known as Cooper pairs. People had written theory papers saying that you could tie the behavior of the fermionic gas to concepts in high-temperature superconductors. It’s difficult to study these superconductors because they’re solid materials whose properties you can’t tune very easily. With the condensate, you can just change its parameters by tuning a knob. Insights from studying the condensate could help us better understand superconductors.
Your adviser was Debbie Jin, a pioneering experimentalist in the study of quantum gases, who passed away in 2016 at age 47. What was it like working with her?
She gave me the mentality that in an experiment, you just start, and eventually, you understand a lot more. Exploring was not only allowed, but actually the right way to do things. It was great to see someone who wasn’t afraid to go for it and just see what you can measure. She told me that when you write a paper, you end it understanding a lot more than when you started.
She won the MacArthur Fellowship, also known as the “Genius Grant,” in 2003, while you were her student. Did that affect the day-to-aspect of the lab?
I don’t think so. It may have affected some of the new experiments she was building, but on the experiment I was working on, it was just, “Let’s keep doing cool science.”
From grad school into your postdoc, you shifted from these gases to working with nanometer-sized solid objects, known as nanomechanical objects, which you still study today. Why did you make that shift?
I wanted to feed my interest in building different physical platforms from the ground up. Also, the idea of cooling atoms with lasers had enabled so many new scientific horizons, it was interesting to think about what other objects you could cool with lasers. In laser cooling of atoms, as the atoms move toward the laser, the light pushes on them more and more, slowing them down. That we could push on a solid in a similar way, and it didn’t heat up, but rather cooled down, opened new opportunities.
You also cool atoms in traps known as optical tweezers. What was the motivation for that work?
In all my grad school experiments, I worked with millions of atoms. You can measure something about those million atoms, but you can’t pinpoint one particular atom doing any particular thing. I became interested in how to control single atoms, and their individual degrees of freedom, and broaden their applications.
What is a single degree of freedom?
It could be a single atom. It can also be a vibrating membrane that is 100 nanometers thick. It’s a little funny to call it a single degree of freedom because the object is composed of many atoms. But it’s called that because you study one vibrational mode of it.
I’m working on experiments to control single degrees of freedom more and more, and I study how this control might allow us to solve problems in quantum mechanics. We’ve done experiments looking at how well can you measure the position of an object, or how the recoil from a photon hitting a sensor affects your ability to measure in an interferometer like LIGO [the Laser Interferometer Gravitational-Wave Observatory]. This type of work often leads to a really good sensor. Some of our other work leads to platforms for building up a quantum computer.
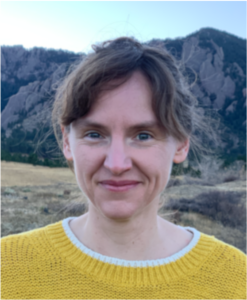
What have you done with these tiny cooled objects?
My group, along with others, showed that you could actually put a membrane of material, similar to a tiny drum, in its quantum ground state. You can think of this drum as a quantum harmonic oscillator, where it vibrates at discrete energy levels. Usually, you can’t see those energy levels because the object has so much thermal energy. But we were able to see the discrete energy levels of the vibration of this drum.
What’s your strategy when something really isn’t working in the lab?
My undergrad advisor said once that working on an experiment can sometimes feel like it’s gone backwards. All of a sudden it doesn’t work anymore, when it was great yesterday. I emphasize to myself and to students that sometimes you have to pivot. Maybe the experiment that you came up with was too hard, and you have to think of another approach to the problem.
How has the pandemic affected the way that you view your work?
The biggest impact on my life is that my daughter’s school was closed for a while. I learned how to teach phonics, and how they teach math, these days. I learned more about being a teacher from teaching part of first grade. It brought me around to how much a first grader doesn’t know. It gave me some perspective about how you teach and explain your work.
What do you like to do outside of physics?
In my younger years, I played violin a lot. These days, my family and I like hiking outdoors. We’ve also planted a variety of trees at our house, and when we travel around the world, I like to identify the trees.
Sophia Chen is a science writer who covers physics, space, and anything involving numbers. Find more of her work at sophurky.com.
Want to recommend a physicist for us to profile? Write to info@1400degrees.org. All interviews are edited for brevity and clarity.